A Laboratory in a box
A focus on plankton
Plankton are among the most diverse groups of organisms on Earth, with millions of known and unknown species drifting in all oceans, lakes, and rivers. Plankton diversity is essential to support the primary production and nutrient cycling in the ocean, and for the functioning and stability of marine ecosystems.
‘All marine life depends on the health of the plankton community.’
However, this diversity is threatened by various human activities which can cause species extinctions, shifts in community composition and function, or loss of ecosystem services. There is, therefore, an urgent need for new technology to improve the quality and accessibility of marine plankton biodiversity data so we can recognize, understand, and adapt to changes in marine biodiversity. The technology exists to measure shapes and sizes of particles in fluids—can this be applied to plankton monitoring?
The Continuous Plankton Recorder survey
Hosted by the Marine Biological Association, the Continuous Plankton Recorder (CPR) survey is a renowned autonomous observing system that operates on basin scales over multiple decades (Fig. 1). By using Ships of Opportunity for scientific research (SOOPs), which sail regardless of scientific involvement, the CPR Survey has a low environmental impact and covers a large geographic area.
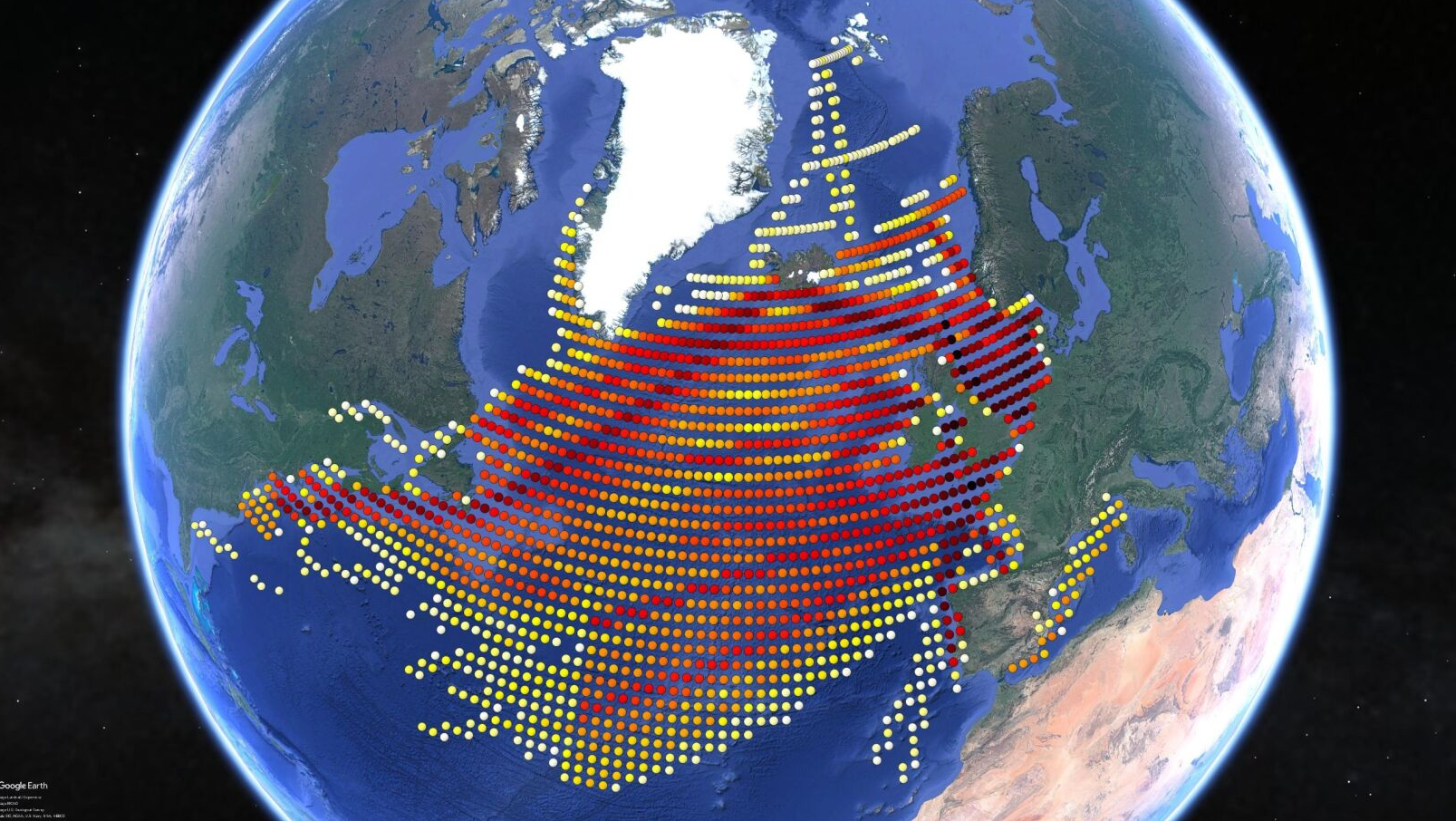
The CPR survey has collected plankton samples using the same methodology since 1958, towing more than 7.2 million nautical miles and covering 20,000 km in the major ecoregions of the North Atlantic every month. The drive for more sophisticated and efficient marine environmental monitoring has led to new technologies and sensors being incorporated into existing infrastructure such as the CPR survey.1
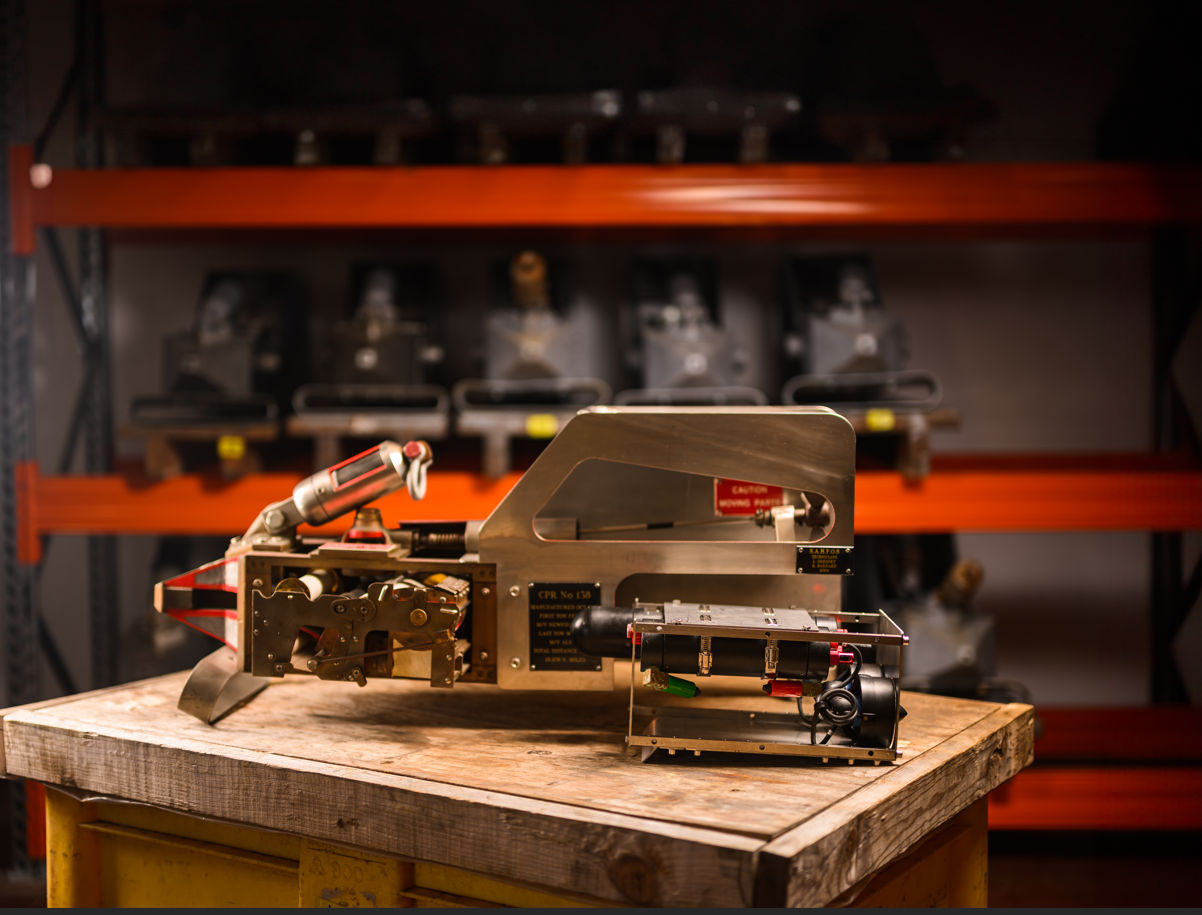
Demonstration unit of an iCPR (Integrated Continuous Plankton Recorder) sampler. The CPR body is cut away to show the internal mechanism, and the iCPR module on the side.
The first Continuous Plankton Recorder was towed by the SS Albatross between Hull, north-east England and Bremen, Germany, on 15 September 1931. The CPR Survey has worked with the commercial shipping industry for many decades and is an established platform, providing a global network of Ships of Opportunity for scientific research.
The CPR sampler (Fig. 2) is towed behind merchant vessels on their regular monthly trade routes across the world’s oceans at around 7–9 m depth. The sampler consists of an outer torpedo-shaped body and an internal removable mechanism. Using a natural silk filtering mesh, the sampler collects plankton along the vessels’ routes and preserves it for analysis by skilled taxonomists.
Sir Alister Hardy conceived the CPR sampler in the early 1920s as a means of gathering plankton from the near surface, to better understand the observed fluctuations in fisheries’ yield. The sampler was designed to be simple to handle and deploy; robust, so it would work virtually every time with minimal maintenance; and inexpensive. A century on, the CPR sampler is undoubtedly the most successful device of its kind ever built, able to routinely go to sea regardless of conditions, collect biological data, and bring it safely back to a laboratory. Trials to improve the sampler have taken place over the decades; however, keeping to the three simple principles has proved challenging, to say the least. For instance, adding sensors to measure key environmental parameters was attempted, but few electronic devices can withstand being tossed overboard at 20 knots (almost 40 km/h).
An Integrated Continuous Plankton Recorder is born
Advances in technology have enabled the long-awaited transformation of the existing sampler into a modern autonomous platform, revolutionizing monitoring capabilities while retaining consistency with the historical data gathered over almost 7 decades. In 2020, the iCPR project was born with a simple mission: ‘use modern sensing technology and introduce Artificial Intelligence (AI) across our existing global plankton observation network’. And, of course, this new platform had to remain simple, robust, and cheap.
The iCPR sampler is equipped with a suite of sensors able to capture the physical performance of the sampler, key environmental variables, and precise digital images of plankton using a bespoke holographic camera (Figs 3 & 4). Fitting neatly inside a traditional CPR sampler (Fig. 3), the iCPR module transforms it into a self-powered platform able to sample anywhere a commercial ship is operating, with—except for deployment and retrieval—no intervention from the crew. Using the flow of water through the sampler body, two impellers generate electricity: an iCPR sampler needs no batteries and can record data over long periods of time.
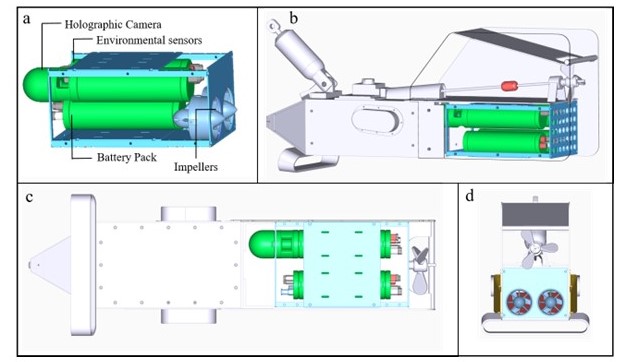
The iCPR sampler monitors the environmental parameters that most strongly influence plankton. Temperature is a primary driver of the physiology of any plant or animal. It also gives us critical information on the structure of the water mass that the sampler is passing through. Salinity indicates oceanographic features such as ocean fronts and gyres. Finally, fluorometers allow us to directly measure the chlorophyll-a present in the environment—an excellent indicator of the productivity of an oceanic water mass.
Taking pictures
An exciting development of iCPR instrumentation is its capacity for in situ imaging through a holographic camera, developed at the University of Plymouth, which enables focused, fine resolution images, even at tow speeds exceeding 20 knots. Automated in situ marine imaging is not new, but it has taken decades for the technology to mature to the point where such imaging systems are reliable. Our iCPR holographic camera is capable of generating hundreds of thousands of images in a matter of hours. See Box.
Box – Digital holography
Digital holography is based on the diffracted light field created by interference from objects in a sample which is illuminated by a laser (see Fig. 5). This results in a longer path-length of diffracted light compared to the undisturbed path. Where the diffracted waves meet the undisturbed waves in phase, they combine to generate an additive (or constructive) wave. When waves interact out of phase, they subtract, reducing or cancelling each other, creating deconstructive waves. This process creates concentric rings of alternating constructive and deconstructive interference, and the wavefront of these patterns is captured by a camera sensor. By using the properties of the original light source as a reference, interference patterns are used to reconstruct the three-dimensional sampling plane, resolving particles at their true position along the axis of the sampling volume. The result is a focused image of particles across the entire sample volume at a good resolution.
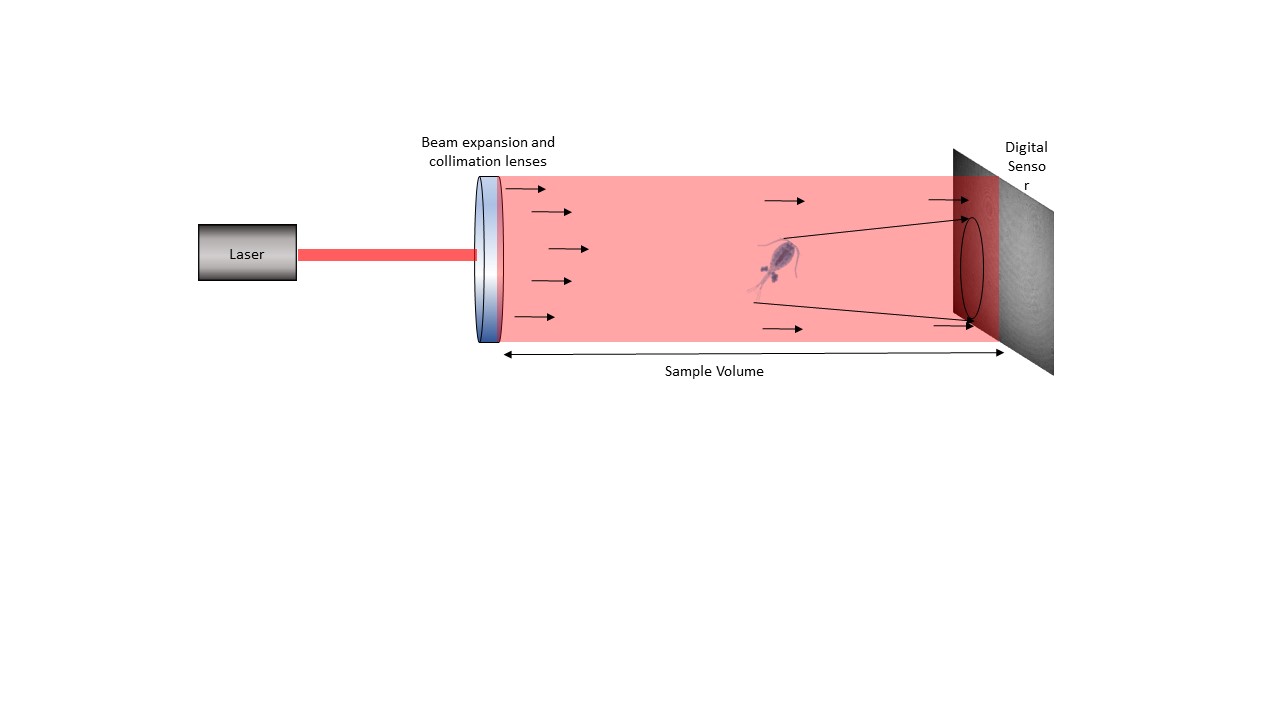
In situ images (i.e. holograms) allow biological contents to be visualized directly from bulk volumes (see Fig. 6) without disintegrating cell structures or disrupting the standard operating methodology of the CPR. At this stage, automated measurements of focused particles are made. In situ digital imaging can provide detailed information on the distribution, abundance, and morphology of plankton species across large areas and scales. It is, therefore, a valuable source of consistent data and information on the status and trends of biodiversity at various scales. Another way to use holographic images is to categorize particles not as species, but on the basis of functional morphology. An AI system to do this is being developed by the CPR.
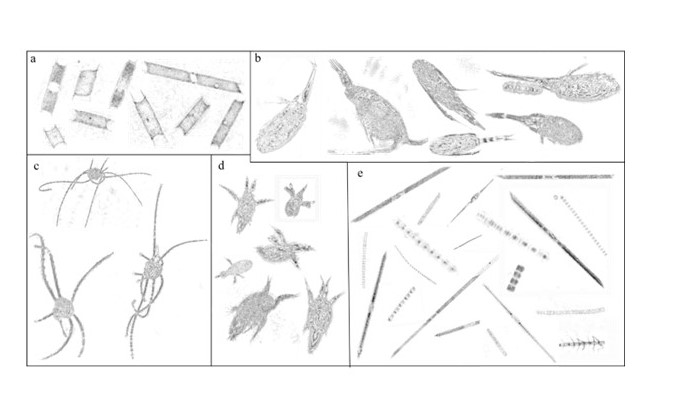
The science of tomorrow, today
The CPR survey is now routinely trialling the iCPR in the Irish Sea (Liverpool–Dublin). In the second half of 2023, we added Tromsø to Svalbard in the Norwegian Sea (see Figs 7 & 8). This route is important from a scientific point of view, as it is where the Atlantic and Arctic Ocean meet, and the temperature of the Arctic has already risen at a rate of almost three times the global average. In November 2023, we sent our first iCPR sampler on our longest transatlantic route, linking Iceland to Newfoundland and Labrador, Canada. This route crosses the transition area between the Labrador Sea and the Atlantic Ocean, as well as the sub-polar gyre, and is an area which has already shown impacts of climate change.
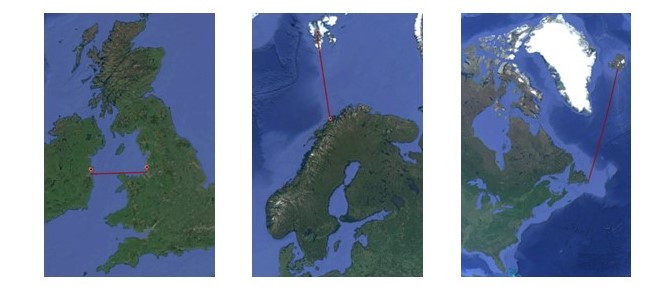
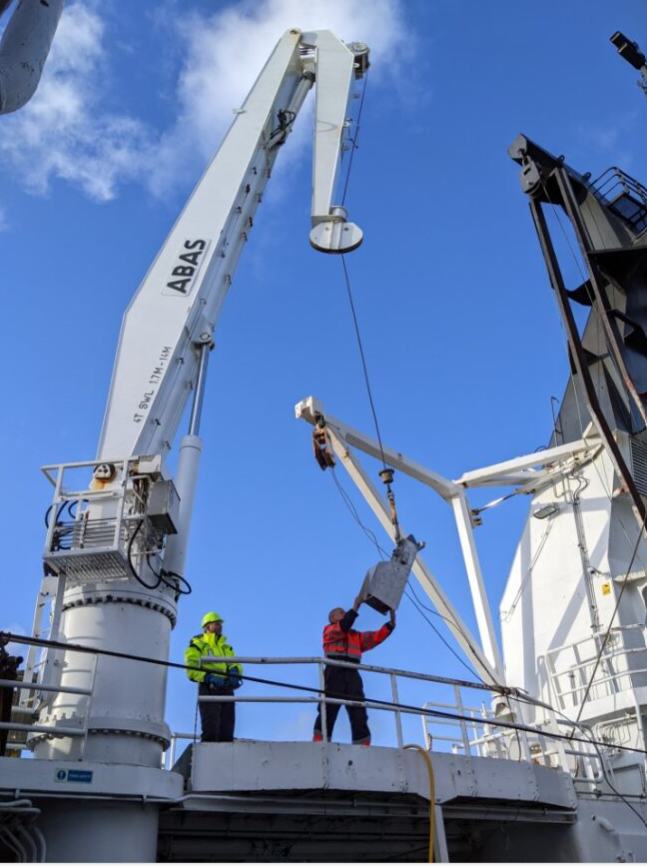
Eventually, all our CPR samplers will be replaced with iCPRs. Towing more than a dozen iCPR samplers every month will enable us to grow our image libraries to train our AI models, optimize our measurement protocols, and deepen our understanding of plankton dynamics and marine diversity. But this large-scale deployment of iCPR samplers comes with challenges. Collecting hundreds of thousands of images per month and supporting data requires the development of advanced computing and processing infrastructure able to analyse images, measure and classify particles, and output information for research and policy.
It is a testament to his vision and practicality that Sir Alister Hardy’s original design has survived virtually unchanged for nearly a century. As technology develops, this robust, mechanical data gathering box will continue to be a sophisticated platform for ocean science of the future.
Pierre Hélaouët (pihe@MBA.ac.uk)

- Dr. Pierre Hélaouët is a senior numerical ecologist and data scientist at the Marine Biological Association (MBA). Pierre led the iCPR project and oversees the continuation of the program. He is specialised in marine planktonic biodiversity and part of the CPR team for more than 15 years. He has also a central role in the MBA’s data team, in charge of developing and disseminating scientific products. using MBA

- Pr. Alex Nimmo Smith is Professor of Marine Science & Technology at the University of Plymouth and is part of the Marine Physics Research Group (MPRG). Alex developed the iCPR module. He has developed several in situ optical systems to directly image particles for measurement and identification and deploys these on autonomous platforms.
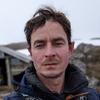
- Robert Camp is the instrumentation and data technician for the Continuous Plankton Recorder (CPR) Survey department of the Marine Biological Association. Rob had a central role in building the iCPR module and is now in charge of supervising the deployment/retrieval of the device as well as its maintenance.
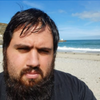
- Kairan Cursons is a PhD student (MBA/Plymouth University). The aim of this PhD is to process plankton images from a range of ex situ and in situ sources to develop a new generation of merged digital image libraries. These libraries will then be used to train AI models (e.g. Convolutional Neural Networks) to automatically identify and classify plankton images through transfer learning.