The fall of the great ocean farmers
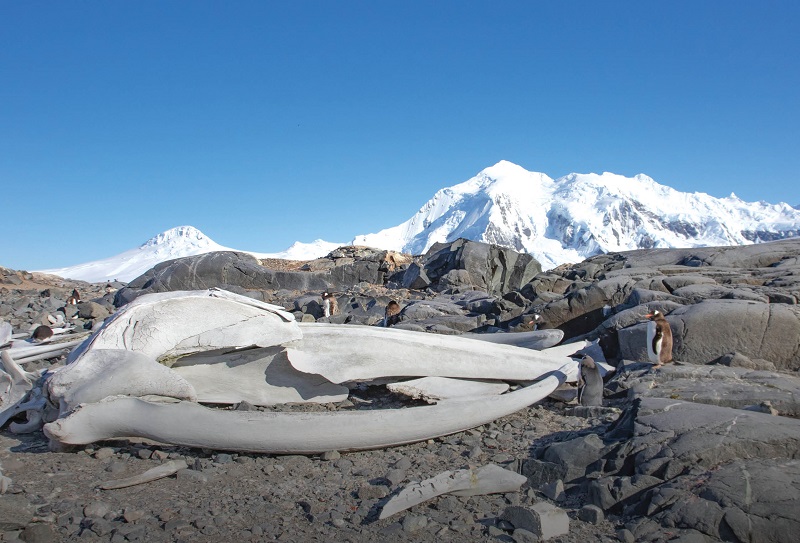
Figure 1. Gentoo penguins around the skull of a blue whale. Port Lockroy, Wiencke Island, Antarctica. © Conor Ryan.
At the turn of the 20th century, all fertilizer ever used was some form of decaying organic matter or animal waste, most often domestic animal manure or seabird guano. The latter was so valuable that increasing scarcity of once-plentiful deposits triggered wars in the mid-19th century. It was clear that humanity could only get so far with natural fertilizer.
In 1908, the German chemist Fritz Haber achieved something remarkable. Under immense pressure and temperature, he broke one of the strongest naturally occurring chemical bonds, namely the triple bond connecting two airborne nitrogen atoms. Haber had artificially created ammonia (NH3) out of thin air—the first step towards synthetic fertilizer.
Haber won the Nobel Prize in Chemistry 1918 for this breakthrough, after collaborating with Carl Bosch, the German engineer who transformed Haber’s laboratory experiment into an industrial-scale process. After the Second World War, synthetic nitrogen fertilizer—produced by the Haber-Bosch Process—became the industrial fertilizer of choice. The proliferation of this fertilizer is the main reason the human population has been able to easily exceed 5 billion and will crest over 10 billion this century.
Biological productivity on most of the Earth, both on land and at sea, is limited by bioavailable nitrogen. However, in some regions, productivity is limited by other elements.
Iron in the Southern Ocean
The Southern Ocean is a marvel for many reasons—its biology, oceanography, and control over global climate. One oddity about this region is a huge pool of nitrogen in its surface waters. In temperate oceans, this vast reservoir of bioavailable nitrogen would stimulate massive blooms of phytoplankton, but here, it goes unused.
This baffling surplus was a mystery for decades until Californian oceanographer John Martin proposed a radical idea. In several papers published between 1988 and 1990, Martin suggested that an iron deficit stymied phytoplankton growth in the Southern Ocean. Initially, the marine science community was sceptical—micronutrients were not known to limit productivity at the scale of entire oceans.
Micronutrients like iron are needed by organisms only in tiny amounts, as compared to macronutrients like nitrogen. When the iron runs out, phytoplankton cannot grow, no matter how much nitrogen or other nutrients are available.
From 1999 to 2009, a number of experiments tested this iron limitation hypothesis directly. The researchers ‘fertilized’ kilometres-wide patches of the Southern Ocean’s surface with dissolved iron—and each time phytoplankton bloomed in response. Unshackled from iron limitation, these phytoplankton rapidly incorporated available nitrogen. The lack of iron explained the abundance of bioavailable nitrogen in these waters. Unfortunately, Martin died before his ‘Iron Hypothesis’ became the paradigm by which we now understand biogeochemical controls on productivity in the Southern Ocean. So why is the Southern Ocean iron limited, even though most of the ocean is not?
The primary source of iron is the Earth’s crust. In temperate regions, airborne dust supplies iron to the open ocean. Indeed, most of the iron in the surface waters of the North Atlantic originates from dust blown off the Sahara Desert. In coastal regions, iron originates from silt present in river outflows, dust released from melting ice, coastal erosion, or by upwelled deep water rich in iron and other nutrients.
The issue is, there is very little land to source dust or silt in the Southern Hemisphere, and land below 60 degrees south latitude is frozen year round. Upwelled deep water and melting seasonal ice near the Antarctic continent and subantarctic islands supply these nearshore regions with iron. But as Martin showed, beyond the continental shelf, iron is almost non-existent in the epipelagic Southern Ocean.
The krill paradox
At the turn of the 20th century, the Southern Ocean was a pristine ecosystem. Once technological advances enabled the exploration of Antarctica and its seas, exploitation was swift. At the time Roald Amundsen, Ernest Shackleton, and others first visited the region, there were one million blue and fin whales in the Southern Ocean. Only 70 years later (roughly a whale’s lifespan), rapid harvesting reduced fin whales by 90 per cent and blue whales by over 99 per cent.
In total, over one million baleen whales were harvested from the Southern Ocean between 1900 and 1970, a total heft that is more than twice the weight of all wild mammals on Earth today. Most of these whales were killed in the Atlantic sector of the Southern Ocean, where they were most abundant. These whaling grounds were approximately 4,000,000 km2; 12 times the size of the United Kingdom. To feed these whales, this region likely supported half a billion tonnes of krill.
Paradoxically, the abundance of krill—the sole food item for Antarctic blue and fin whales—in the former whaling grounds declined by 80 per cent in the three decades immediately following industrial whaling. This puzzled scientists: Prey should increase, not decrease, after predators are exterminated. Moreover, the decimated whales were not sufficiently replaced by other krill predators (for example, seals, seabirds, and fish) to explain the abrupt decline in krill. Climate change is probably in part to blame, but something else was afoot: climate change alone could not explain the rapid decline of such an abundant animal in the near total absence of its main predators.
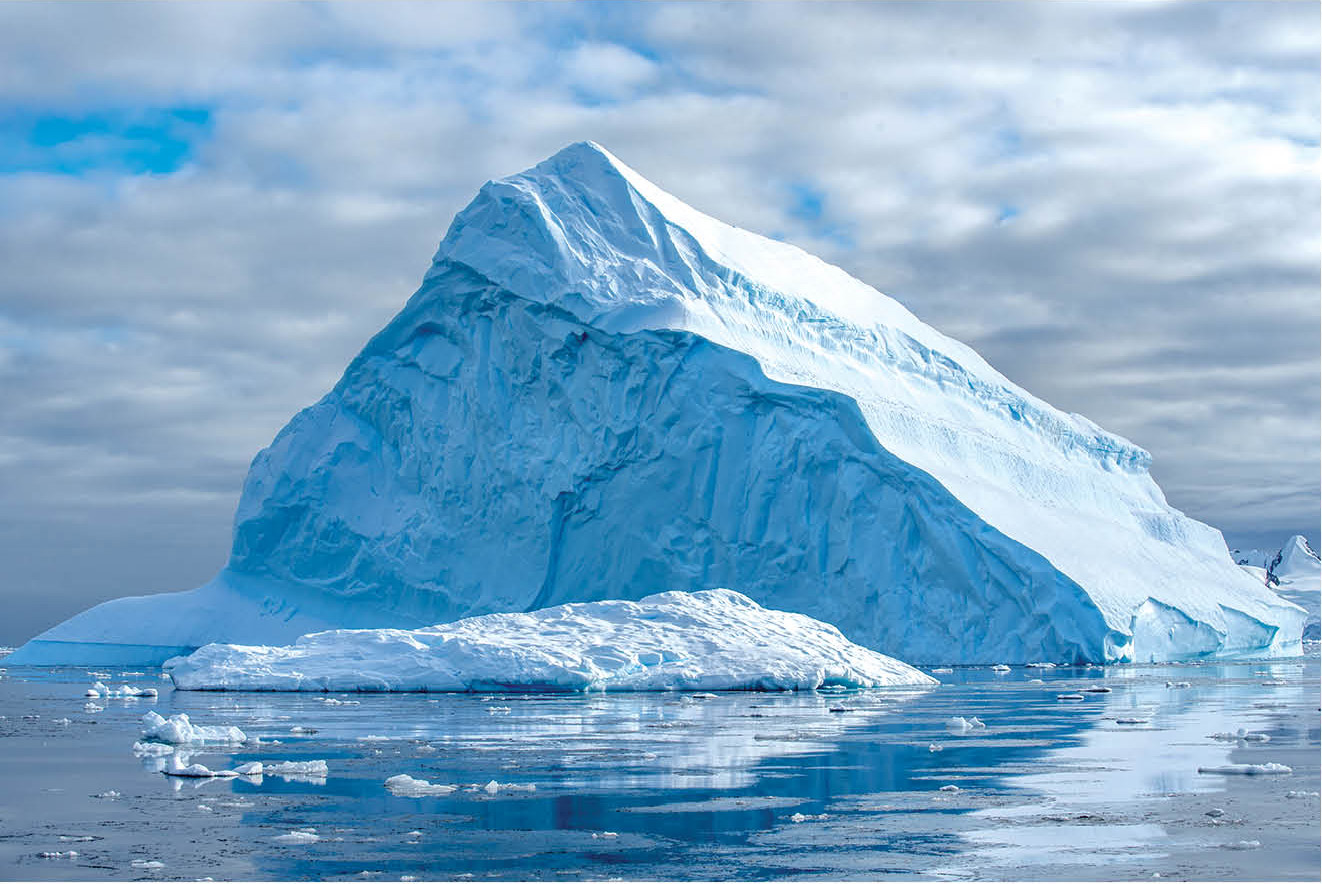
Figure 2. Icebergs in the Southern Ocean trap and transport essential micronutrients, including iron. © Matthew Savoca.
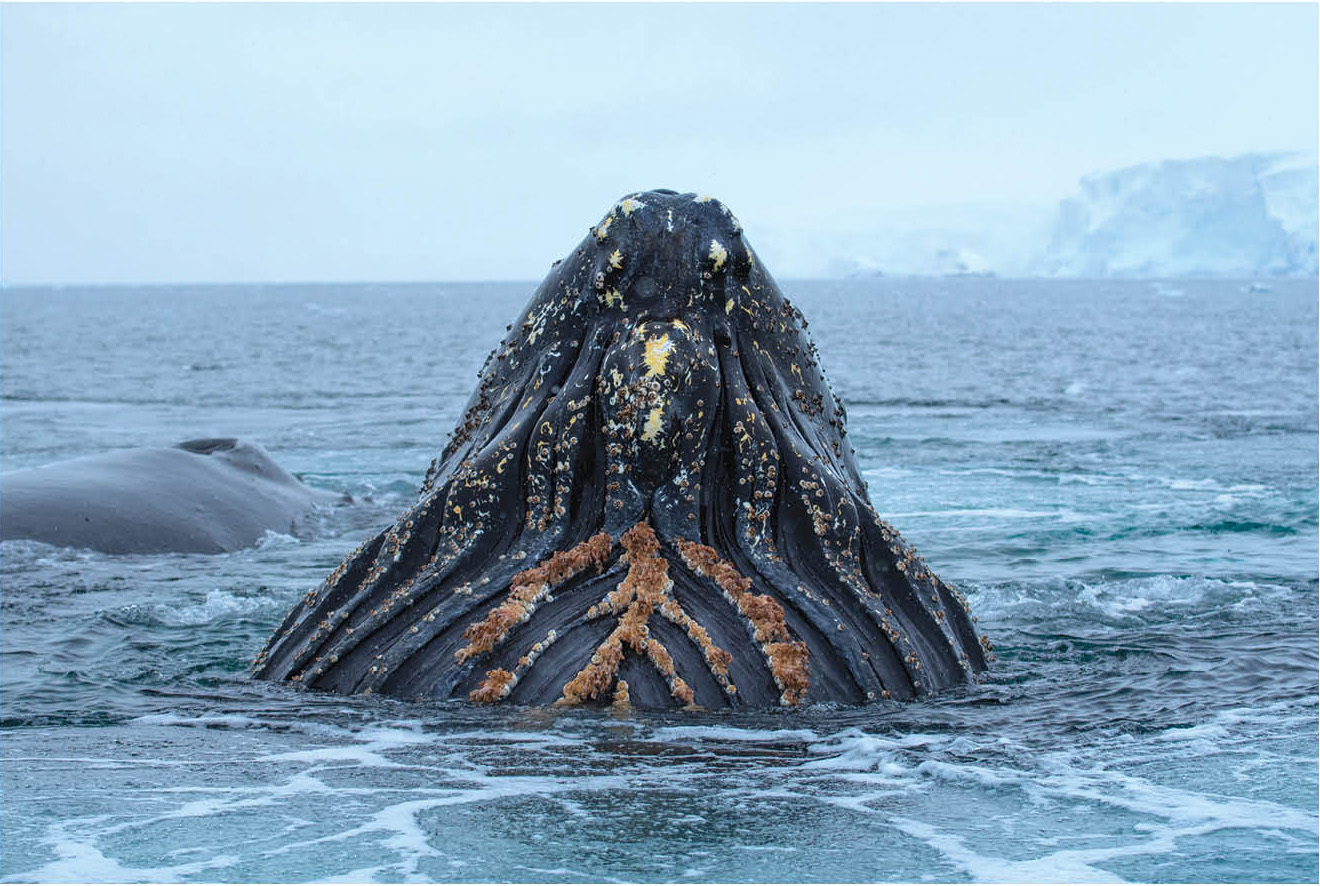
Figure 3. Over one million baleen whales were harvested from the Southern Ocean between 1900 and 1970. © Matthew Savoca.
Whales as farmers
This paradox remained unresolved for three decades until two oceanographers, Victor Smetacek and Stephen Nicol, posed an intriguing possibility: what if the whales were farmers? Of course, they didn’t phrase their idea quite that way. Rather, they said, what if the whales seeded the krill pastures with limiting iron that allowed phytoplankton to bloom, which, in turn, supported much larger populations of krill? And how did the whales fertilize phytoplankton? The same way humans had fertilized fields for millennia: poop.
Studies from around the world have since convincingly demonstrated that whale excreta is highly enriched in nutrients including nitrogen, phosphorus, manganese, and iron. In particular, the concentration of iron in whale feces is approximately ten million times higher than that of Southern Ocean seawater. Iron is so limiting in this region that the addition of one gram of iron in whale feces could seed several kilograms of carbon incorporated into phytoplankton—a potent fertilizer indeed. The fertilization effect of whale feces would have been particularly pronounced before whaling.
At the turn of the 20th century, baleen whales ate twice as much Antarctic krill as is currently estimated to exist. For decades, scientists have been debating the extent of krill production before the whaling era. These guesses span a vast range, from 500 to 2,000 million tonnes. Research my colleagues and I recently published implies that the higher end estimates are likely to be true.
Whales in the Southern Ocean maintained a standing stock of krill with the same biomass that humans maintain cattle on a global scale today. In other words, whales were the greatest farmers on Earth only 100 years ago.
The scale of the whales’ ‘farming’ cannot be overstated, and it had to be immense. In addition to being massive, baleen whales have a surreal rate of cell division and absolute growth. A blue whale is sexually mature by 7 years old, at which point it has achieved around 70 per cent of its final weight—from a single-celled zygote to a 70-tonne animal in less than 10 years.
In the early whaling days, the time between calves for female blue and fin whales was 2–3 years. That is exceptionally fast for an animal the size of an aeroplane. To adapt to variations in food supply, baleen whales likely altered their reproductive rates, such as by increasing their time between calves. With abundant food, more females would be expected to be reproductively active (pregnant or raising young), while food shortage would have the opposite effect. While ecological theory suggests this would be true, these relationships are difficult to verify, due to the difficulty of studying these now-rare whales today.
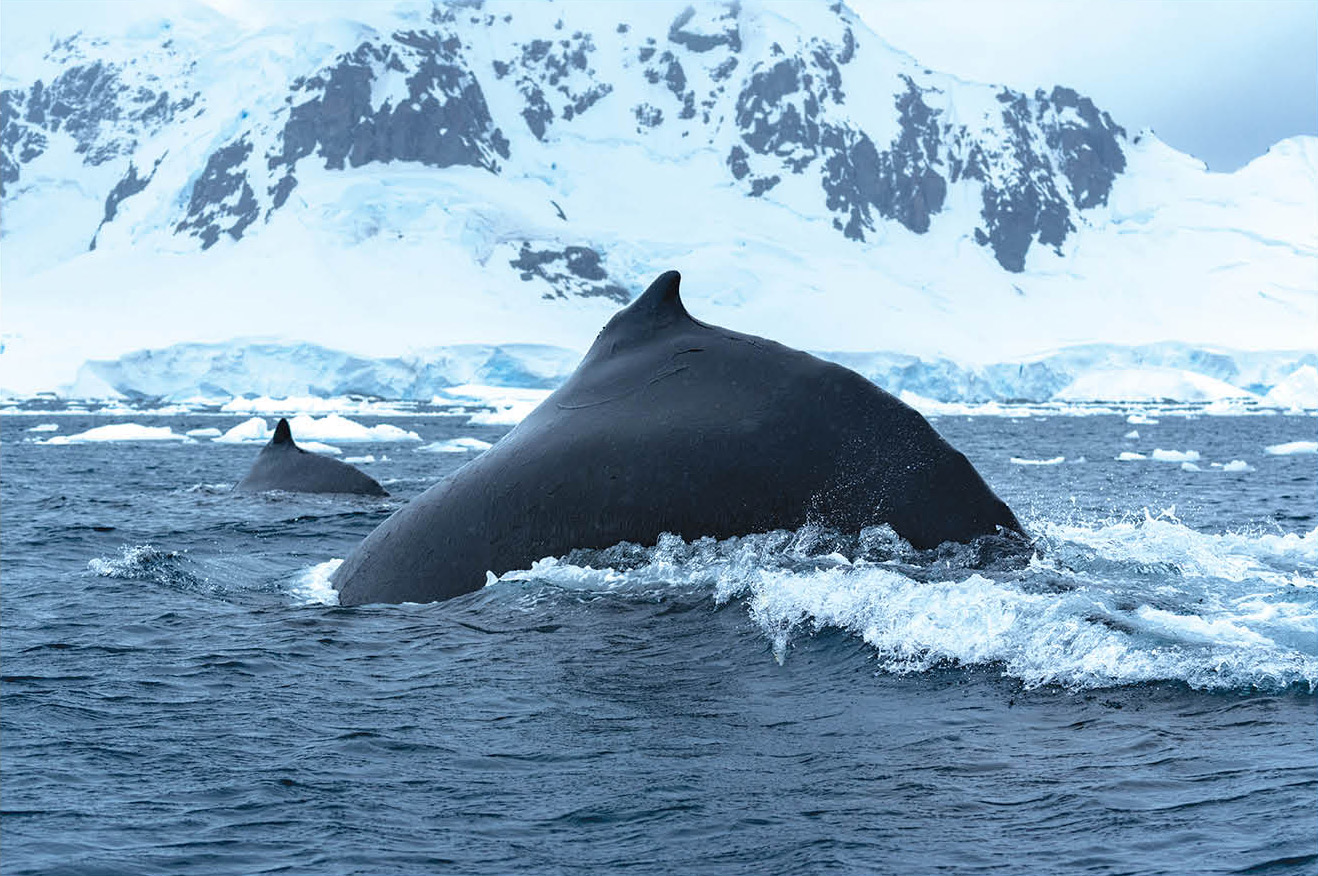
Figure 4. Humpback whale numbers in the Southern Ocean have returned to pre-exploitation levels. © Matthew Savoca.
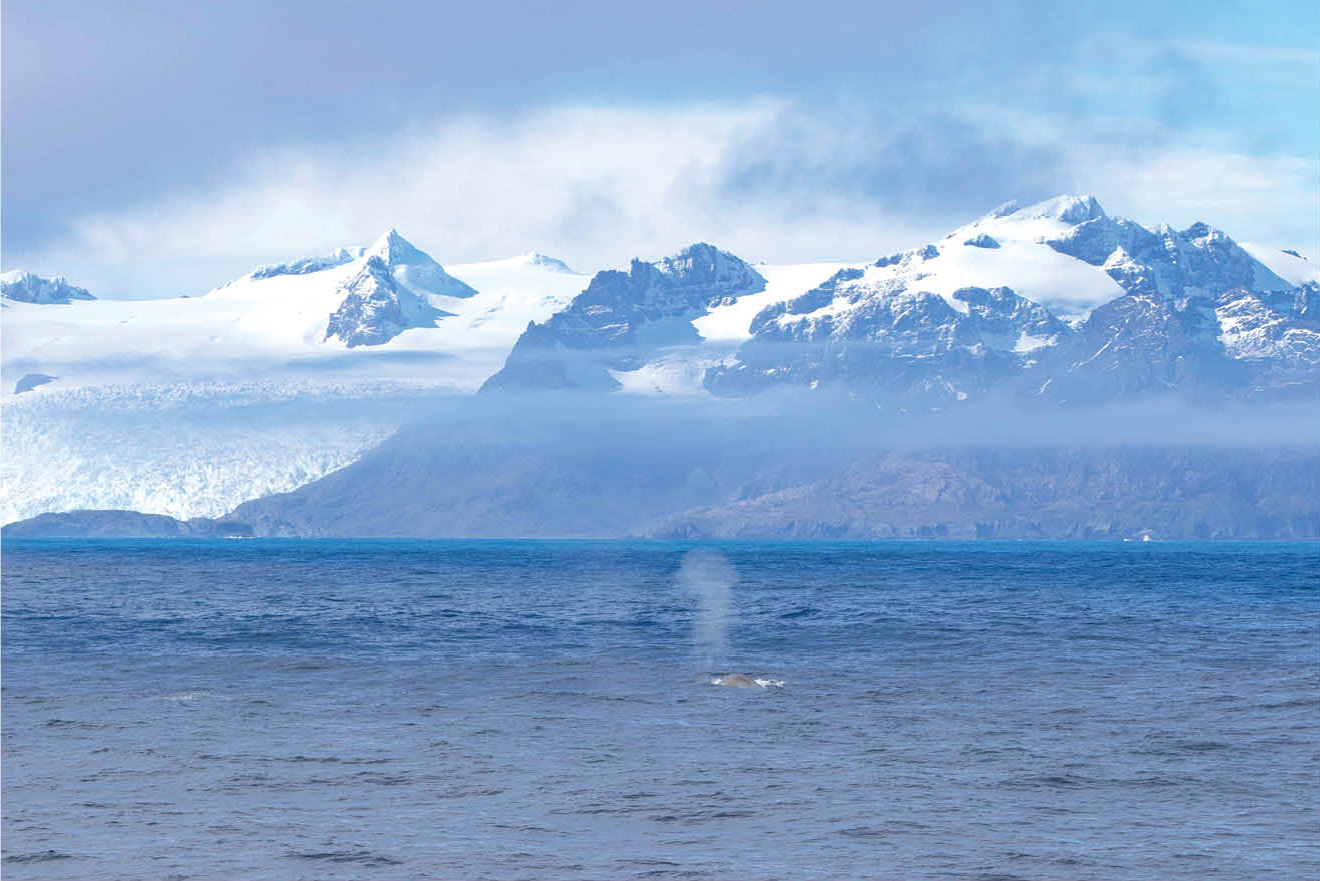
Figure 5. Blue whale mother and calf. Annekov Island, South Georgia. © Conor Ryan.
Whale recoveries
In a paper published in 2004, Trevor Branch, a quantitative ecologist at the University of Washington, suggested that Antarctic blue whales should be recovering well. However, nearly 20 years later, and 60 years after we stopped hunting them altogether, this has still not come true. Why?
Branch and his co-authors used reproductive rates from the whaling days—the only data available—to parameterize their model. This was behind their key finding of a large rate of population increase (5–10 per cent per year, compounding) in Antarctic blue whales. However, their underlying assumption had a critical flaw. They did not consider (as no one did at the time) that the krill need the whales just as much as the whales need the krill. Without the whales, krill abundance declined, which has constrained the recovery of the whales. Antarctic blue and fin whales are stuck at less than 10 per cent of their historical abundances.
Not all whales are struggling. Humpback whales in the Southern Ocean have rebounded to pre-exploitation numbers after being similarly wiped out by whaling. This is likely due to their ability to use habitats including small bays and narrow straits, as well as the open ocean. Conversely, blue and fin whales need open ocean habitat and massive, dense krill swarms to thrive. These pelagic regions remain extremely iron limited and krill depleted to this day. At present, the total population of krill on former whaling grounds is 60 million tonnes. Our calculations suggest that pre-whaling populations of blue and fin whales consumed 100 million tonnes of krill annually in this region.
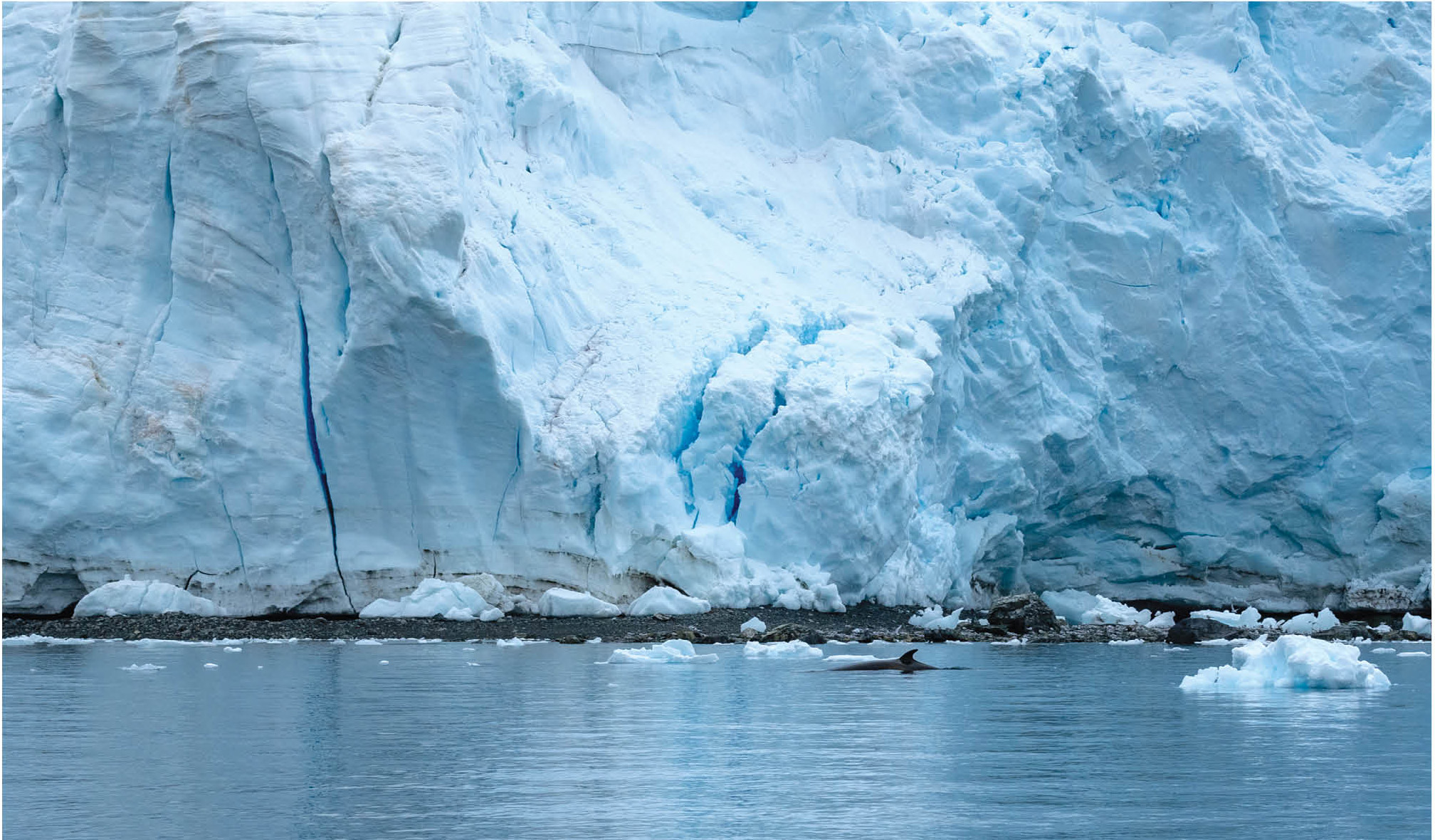
Figure 6. Antarctic minke whale with a dramatic backdrop of ice. © Matthew Savoca.
The often-listed threats to large whales are entanglement in fishing gear, ship strike, and pollution (e.g. acoustic, microplastic, and chemical). However, these stressors have only minor effects on whales in the Southern Ocean at present. The main hindrances to their recovery are prey limitation and climate change.
Recovery plans for Southern Ocean whales must have an ecosystem-wide approach. Mitigating climate change, reducing krill fishing, and creating or expanding marine protected areas (such as the one currently under consideration in the Western Antarctic Peninsula) would be a good start. More radical proposals, such as fertilizing the former whaling grounds with iron to rebuild the krill stock, should be considered.
While it is easy to despair in the downfall of Antarctic krill and baleen whales, I see hope in the sliver of both that remain. This ecosystem, its major players, and the oceanic-climate regime under which the pre-whaling Southern Ocean evolved, still exist. If we marshal our collective creativity with aggressive and relevant recovery actions, we stand a chance to bring back the great ocean farmers.
Matthew Savoca
(msavoca13@gmail.com), Postdoctoral researcher, Hopkins Marine Station, Stanford University.
Matthew Savoca: @DJShearwater
Conor Ryan: @whale_nerd
conorryan.photography
Supporting statement:
Matthew Savoca is supported by a Postdoctoral Research Fellowship from the National Science Foundation, The National Geographic Society, and MAC3 Impact Philanthropies.
Further reading
Branch, T. A., Matsuoka, K. and Miyashita, T. (2004). Evidence for increases in Antarctic blue whales based on Bayesian modelling. Marine Mammal Science 20: 726–754.
Martin, J. H., Gordon, R. M. and Fitzwater, S. E. (1990). Iron in Antarctic waters. Nature, 345: 156–158.
Nicol, S., Bowie, A., Jarman, S., Lannuzel, D., Meiners, K. M. and Van Der Merwe, P. (2010). Southern Ocean iron fertilization by baleen whales and Antarctic krill. Fish and Fisheries 11: 203–209.
Savoca, M. S., Czapanskiy, M. F., Kahane-Rapport, S. R., Gough, W. T., Fahlbusch, J. A., Bierlich, K. C., Segre, P. S., Di Clemente, J., Penry, G. S., Wiley, D. N., Calambokidis, J., Nowacek, D. P., Johnston, D. W., Pyenson, N. D., Friedlaender, A. S., Hazen, E. L. and Goldbogen, J. A. (2021). Baleen whale prey consumption based on high resolution foraging measurements. Nature 599: 85–90.
Smetacek, V. (2008). Are declining Antarctic krill stocks a result of global warming or of the decimation of the whales? Pp. 46–80 in C. M. Duarte, ed. Impacts of global warming on polar ecosystems. Fundacion BBVA, Madrid, Spain.